Genomic insights into inherited bone marrow failure syndromes: a single-center clinical study
Abstract
Aim: Inherited Bone Marrow Failure Syndromes (IBMFS) are increasingly diagnosed in both pediatrics and adults presenting with cytopenias, myelodysplastic syndrome, and acute leukemia due to increased awareness among providers. Given the multi-organ involvement in IBMFS, the referrals for genetic testing have extended to individuals with pulmonary fibrosis, liver cirrhosis, and concerns for cancer predisposition in other solid tumors. The purpose of this study is to highlight the real-world data of genetic testing in an Inherited Bone Marrow Failure clinic and the challenges faced.
Method: Patients who were referred to the Medical Genetics and Genomics Outpatient Clinic for evaluation of a suspected underlying IBMFS at the University of Iowa from January 2023 to September 2024 were enrolled in this study. Clinical phenotypes, reasons for referrals, and genetic testing results were recorded and analyzed.
Result: A total of 16 individuals from 15 families were enrolled in this study during this period. Clinical-grade genetic testing yielded a diagnosis in 50% of the patients, with a predominance of patients diagnosed with a telomere biology disorder.
Conclusion: Early diagnosis of an underlying IBMFS is important to reduce mortality and morbidity. Genomic testing is crucial to provide an accurate diagnosis and appropriate management, including donor selection when hematopoietic stem cell transplant is a treatment option.
Keywords
INTRODUCTION
Inherited bone marrow failure syndromes (IBMFS) are a group of rare genetic disorders due to germline pathogenic variants associated with hematopoiesis[1-3], telomere biology[4,5], ribonucleic acid (RNA) maturation and processing[6], ribosome biogenesis[7,8], and deoxyribonucleic acid (DNA) repair and cellular maintenance pathways[9]. Overall, the estimated incidence of IBMFS is approximately 65 per million live births[10]. The phenotypic heterogeneity in IBMFS and variability in disease penetrance results in significant overlap in clinical and laboratory findings in various IBMFS and other genetic disorders[11-15]. Hematological abnormalities, such as uni-lineage or multi-lineage cytopenias, are the predominant clinical features across all IBMFS and usually lead to the patient seeking medical attention. As some of these IBMFS have an increased risk of developing myelodysplastic syndrome, acute myeloid and lymphoid leukemias, as well as other solid tumors[16-20], they may be diagnosed later in life by their primary hematology oncologist. Extra-hematopoietic features are diagnosed earlier in life when patients present with multiple congenital anomalies, dysmorphism, developmental delays, skeletal dysplasia or short stature[14], while adults may present with recurrent infections, idiopathic pulmonary fibrosis or even cryptogenic liver cirrhosis[12]. Although most IBMFS demonstrate classical Mendelian inheritance consistent with autosomal recessive, autosomal dominant, and X-linked patterns, a positive family history may be absent in up to 40% of patients that harbor a germline pathogenic variant in genes associated with IBMFS[21].
Despite all these complexities in the clinical setting, the rapid development of next-generation sequencing (NGS) has accelerated gene discovery in the setting of IBMFS[22-27]. The advent of massively parallel sequencing has not only revolutionized biomedical research but has now become more accessible in the clinical sector due to rapid turnaround time at a more affordable cost[28]. This has led to various consortiums and professional bodies recommending NGS as an early diagnostic test in patients evaluated for IBMFS[29-31]. The classification criteria of hematological malignancies have evolved to incorporate NGS testing for both tumor profiling using somatic panels as well as defining the co-existence of any germline variants that predispose to the development of malignancies, which includes genes associated with various IBMFS[32-35].
While the progress in genomic diagnoses has been exponential, it is a double-edged sword. The current NGS technique is unable to differentiate between clonal hematopoiesis (CH) caused by somatic mutations within the blood and germline variants seen in IBFMS[36,37]. The risk of a false positive result due to the presence of CH and that of a false negative result due to a rare phenomenon known as somatic revertant genetic rescue[38] are both problematic. This understanding has resulted in the recommendation of NGS testing on cultured skin fibroblasts obtained through a skin punch biopsy as the optimal sample source for determining the presence of germline variants[39]. Additional challenges include the different NGS methodologies used in different cohorts with variable numbers of genes evaluated and the interpretation of variants of unknown significance (VUS) or genes of uncertain significance (GUS)[36].
The clinical importance of an early and accurate diagnosis of IBMFS cannot be over-emphasized. Early identification of patients with IBMFS avoids the use of immunosuppressive therapy (IST), which is not only unhelpful in this group of patients but has the risk of causing harm through cytopenia-related complications and delay in appropriate management[12]. Mortality and morbidity have been shown to improve in patients with IBMFS who undergo hematopoietic stem cell transplantation (HSCT)[40] through the selection of an appropriate donor, conditioning regimen[41], and post-HSCT surveillance[42-47]. The genomic classification of IBMFS not only impacts the patient’s own health and reproductive choices but also influences the health of their immediate relatives. In this study, we want to highlight the novelty of our approach, where the use of exome sequencing in younger patients revealed dual diagnoses in a subset of these patients.
METHODS
Study design and patient population
All patients referred to the Division of Medical Genetics and Genomics for evaluation of an underlying IBMFS from January 2023 to September 2024 were enrolled in this study. Indications for the referral included cytopenia(s) suspicious of an underlying IBMFS, personal and/or family history of hematological malignancy, solid tumor or extra-hematopoietic organ dysfunction associated with IBMFS, personal history of pre-existing cytopenia(s), and abnormal genetic test results in genes related to IBMFS.
Germline genetic testing was performed on peripheral blood or extracted DNA from cultured skin fibroblasts in patients presenting with cytopenias, hematological malignancies, and those who had undergone post-hematopoietic stem cell transplantation. Clinical-grade genomic testing was performed either through targeted next-generation sequencing (NGS) panels or exome sequencing (ES) based on the proband’s clinical phenotype and relevant family history.
Additional variant curation was performed manually if the results returned with a variant of uncertain significance (VUS) based on the American College of Medical Genetics and Genomics and Association for Molecular Pathology (ACMG/AMP)[48] and ClinGen[49] guidelines. Clinical phenotype and ancillary test results, such as telomere lengths measured using the flow cytometry-fluorescent in situ hybridization (Flow-FISH)[50] methodology, were used to annotate these VUS.
RESULTS
A total of 16 individuals from 15 families were included in the analysis for this study. Patient demographics are summarized in Table 1. Our results identified eight individuals with a telomere biology disorder (TBD) (50%). Interestingly, four patients (25%) had dual diagnoses. Two of these patients had multiple congenital anomalies and global developmental delays. An exome sequencing was performed and revealed two rare genetic disorders in each individual, which is summarized in Table 2. Two individuals from the same family (individuals 15 and 16) were diagnosed with hereditary breast and ovarian cancer syndrome (HBOC) and TBD due to a family history of breast cancer and a personal history of B cell acute lymphoblastic leukemia in individual 16. The telomere length measurement was normal in individual 16 using the flow-FISH methodology (summarized in Table 2).
Demographics of patients evaluated for inherited bone marrow failure syndromes
Demographics | N (%) |
Age, years Mean (range) | 28 (3-61) |
Biological sex Male (%) Female (%) | 8 (50) 8 (50) |
Indications for genetic evaluation Abnormal genetic test results in genes associated with IBMFS Personal history of a hematological malignancy Family history of malignancies (hematological and solid tumors) Personal and family history of hematological malignancy Personal history of a hematological malignancy AND family history of solid tumor (s) Personal history of cytopenia(s) Personal history of hematological malignancy AND pre-existing cytopenia Personal history of cytopenia AND family history of extra-hematopoietic involvement | 3 (18.8) 4 (25.0) 1 (6.3) 1 (6.3) 1 (6.3) 2 (12.5) 3 (18.8) 1 (6.3) |
Dual diagnoses Noonan Syndrome and TBD DBA and Smith-Lemli-Opitz Syndrome HBOC and TBD | 1 (6.3) 1 (6.3) 2 (12.5) |
Genetic tests performed Karyotype Chromosomal microarray Fragile X analysis Targeted NGS panel Exome sequencing (singleton) Exome sequencing (trio) | 1 (6.3) 3 (18.8) 1 (6.3) 15 (93.8) 2 (12.5) 2 (12.5) |
Ancillary tests Telomere length measurements by flow-FISH methodology Chromosomal breakage analysis Erythrocyte adenosine deaminase 7 dehydrocholesterol | 8 (50.0) 4 (25.0) 2 (12.5) 1 (6.3) |
Clinical outcomes Alive Deceased | 14 (87.5) 2 (12.5) |
Patients with dual genetic diagnoses and clinical phenotypes
Clinical phenotype | Individual 1 | Individual 3 | Individual 15 | Individual 16 |
Age at diagnosis (years) | 3 | 24 | 46 | 20 |
Global developmental delays | + | + | - | - |
Failure to thrive requiring G-tube feeding | + | + (IUGR) | - | - |
Cardiac anomaly | Atrial septal defect with borderline right ventricular enlargement | Vascular ring | - | - |
Neurological anomaly | Central hypotonia Myoclonic epilepsy | - | - | - |
Ophthalmology | Bilateral esotropia and hyperopia | - | - | - |
Otolaryngology | - | Pierre Robin Sequence with micrognathia and cleft palate | - | - |
Endocrinology | - | Short stature | - | - |
Renal anomaly | Left renal stone | - | - | |
MRI brain | Prominent supratentorial ventricular system | - | - | - |
Genetic tests | ||||
Chromosomal microarray | Normal male array | Normal female array | - | - |
Targeted NGS panel | - | COL2A1, COL11A1: Negative | • Heterozygous PV in BRCA1 (c.1874_1877dupTAGT, p.Val627Serfs*4) • Heterozygous PV in RTEL1 (c.897del, p.Phe299Leufs*10) • Heterozygous LPV in TNFRSR13B (c.542C>A, p.Ala181Glu) | |
Exome sequencing | Singleton: • Heterozygous, LPV in LZTR1 (c.2011_2012del, p.L671Vfs*3) • Hemizygous, VUS in DKC1 (c.472C>T, p.R158W) • Heterozygous VUS in JAG1 (c.2687_2688delinsCT, p.W896S) | Trio: • Heterozygous, de novo PV in RPL5 (c.46_47del, p.Y16Pfs*5) • Compound heterozygous in DHCR7 (LPV maternally inherited, c.89 G>C, p.G30A0; LPV paternally inherited, c.1087C>T, p.R363C) | - | - |
Ancillary tests | Telomere lengths (flow-FISH): 1st percentile | Erythrocyte adenosine deaminase levels elevated at 1,443 mU/g Hb 7DHC and 8 DHC levels mildly elevated at 6.7 and 4.2 mg/L, respectively | - | Telomere lengths (flow-FISH): normal |
Final diagnosis | (1) Noonan Syndrome (2) TBD | (1) DBA (2) SLOS | (1) HBOC (2) TBD |
Nine patients had genomic DNA extracted from cultured skin fibroblasts and only one patient had repeated culture failure (11%). This patient had germline genetic testing on peripheral blood during remission from her underlying diagnosis of acute myeloid leukemia.
Only one patient had a negative genetic test result. Two patients were found to have copy number variants (CNV). Variants in 22 genes were detected among those with an abnormal test result. Recurrent mutations were seen in the TERC, TERT, and RTEL genes and diagnostic for TBD. A total of 26 variants were identified in this patient cohort. Missense variants accounted for the majority of variants, with 20 identified (77%). The second most frequent variants found were those constituting frameshift or insertion/deletions (indels) variants, with 6 identified (23%). Lastly, 2 non-coding variants were identified (7%). Overall, the diagnostic yield was approximately 50% (8/16 patients). A summary of the diagnostic yield and number of variants detected per gene can be found in Figure 1. Eight patients had at least one VUS detected on clinical-grade genetic testing (50%), with one individual having 5 VUS reported. One patient (individual 8) was given the diagnosis of TBD due to short telomeres (1%ile) with myelodysplastic syndrome and pulmonary fibrosis and remains genetically uncharacterized. Another patient (individual 2) received a clinical diagnosis of DBA and remains genomically uncharacterized as well. Individual 12 was found to have a novel TERC variant (n.116C>G) and reclassified from a VUS to a likely pathogenic variant (LPV) based on the American College of Medical Genetics and Genomics and the Association for Molecular Pathology (ACMG/AMP) guidelines for variant interpretation[48], given his clinical presentation of hypoplastic myelodysplastic syndrome with short telomere length of less than the first percentile using the flow-FISH methodology (PS3). These results are summarized in Table 3.
Gene variants detected through clinical testing
Individual | Phenotype | Gene | HGVS coding | HGVS protein | Ancillary tests | Variant type | ACMG/ClinGen classification | Annotation | Final variant interpretation |
1 | Noonan Syndrome | LZTR1 | c.2011_2012del | p.L671Vfs*3 | Del | PS4 (PM2 met) PVS1 (null variant in a gene where LOF is a known MOD) PM2 | NM_006767.4 Chr22-21350100 ACT>A Type: Frameshift indel | PV | |
TBD | DKC1 | c.472C>T | p.R158W | TL: < 1%ile (FF) | SNV | PM2 PP3 PP2 PS4 | NM_001363.5 chrX-153995295 C>T Type: Missense REVEL: 0.68 (Supp Path) | LPV | |
JAG1 | c.2687_2688delinsCT | p.W896S | Indel | PM2 PP2 | NM_0000214.3 Chr20-10622336CC>AG Type: Missense | VUS | |||
2 | DBA | ERCC4 | 376 bp duplication of exon 11 on ERCC4 | eADA elevated: 1,520 mU/gHb | CNV | 1A (0.00) 2I-K (+0.30) 2L (0.00) 3A (0.00) | chr16:14,045,201-14,045,576 ERCC4: NM_005236 (pLI:0) | VUS | |
3 | DBA | RPL5 | c.46_47del | p.Y16Pfs*5 | eADA elevated: 1,443 mU/g Hb | Del | PS4 PVS1 PM2 | NM_000969.5 Chr1-93298986 Type: Frameshift indel | PV |
SLOS | DHCR7 | c.89G>C | p.G30A | 7DHC elevated: | SNV | PM3 PP1 PM1 PP2 PM5 PP3 | NM_001360.3 Chr11-71155910C>G Type: Missense REVEL: 0.78 (Mod Path) | PV | |
c.1087C>T | P.R363C | SNV | PM1 PP2 PM2 PP3 PP5 | NM_001360.3 Chr11-71146762G>A Type: Missense REVEL: 0.7 (Supp Path) | LPV | ||||
4 | TBD | TERC | 86.3kb deletion on chromosome 3q26.2 which involves 4 genes CLDN11, MECOM, SLC7A1A, and TERC. This deletion encompasses the whole of panel gene TERC and exon 1 of transcript variant 4 (also known as MDS1/EVI1) of panel gene MECOM | TL: < 1%ile (FF) | CNV | 1A (0.00) 2A-E (+0.90) 3A (0.00) | chr3:169,381,022-170,244,785 MECOM (exonic 1): NM_004991 (pLI:1) SAMD7: NM_001304366 (pLI:0) TERC: NR_001566 (pLI:N/A) CLDN11: NM_005602 (pLI: 0.91) SLC7A14 (exonic 2-8): NM_020949 (pLI: 0.02) | LPV | |
5 | TBD | TERT | c.2794G>A | p.Gly932Ser | TL: 1%ile (FF) | SNV | PP3 PM2 PP2 | NM_198253.3 Chr5-1264568C>T Type: Missense REVEL: 0.95 (Strong Path) | LPV |
RMRP | n.-25_-12dup | Non-coding | PM3 PS3 PM2 BP7 | NR_003051.3 chr9-35658026 C>CTTCACAGAGTAGTA | LPV | ||||
6 | TBD | TERT | c.1195_1210dup | p.Pro404Glnfs*140 | Not done | Dup | PVS1 PM2 | NM_198253.3 chr5-1293790 G>GGGCACTGCGCGTGGTT Type: Frameshift indel | LPV |
KMT2A | c.11390A>G | p.Asn3797Ser | SNV | PM2 PP2 | NM_001197104.2 chr11-118390740 A>G Type: Missense Final: VUS | VUS | |||
7 | JMMOL | Negative | N/A | ||||||
8 | TBD | MPL | c.1102G>T | p.Val368Leu | 1%ile (FF) | SNV | BP6 | NM_005373.3 chr1-43812237 G>T Type: Missense REVEL: 0.35 (Uncertain) | VUS |
SLX4 | c.996G>T | p.Gln332His | SNV | PM2 BP4 | NM_032444.4 chr16-3651147 C>A Type: Missense | VUS | |||
9 | Thrombocytopenia | CEBPA | c.1009A>T | p.T337S | SNV | PM2 PM1 BP4 | NM_004364.5 chr19-33792312 T>A Type: Missense REVEL: 0.08 (Mod Benign) | VUS | |
ELANE | c.490G>C | p.Gly164Arg | SNV | PM1 PP2 PM2 BP6 | NM_001972.4 chr19-855687 G>C Type: Missense REVEL: 0.35 (Uncertain) Final: VUS | VUS | |||
RTEL1 | c.2600C>T | p.Pro867Leu | TL:Normal (FF) | SNV | PM2 BP4 | NM_001283009.2 chr20-62323138 C>T Type: MIssense REVEL: 0.01 (Strong Benign) | VUS | ||
SLX4 | c.3139G>A | p.Gly1047Arg | SNV | PM2 BP4 | NM_032444.4 chr16-3640500 C>T Type: Missense REVEL: 0.01 (Strong Benign) | VUS | |||
TUBB1 | c.1279G>A | p.Asp427Asn | SNV | PM2 | NM_030773.4 chr20-57599761 G>A Type: Missense REVEL: 0.4 (Uncertain) | VUS | |||
10 | AML | LIG4 | c.2525C>A | p.A842D | SNV | BS1 BS2 BP6 | NM_206937.2 chr13-108861092 G>T Type: Missense REVEL: 0.4 (Uncertain) | LB | |
11 | AML | BLM | c.4163C>T | p.Ala1388Val | SNV | PM2 BP4 | NM_000057.4 chr15-91358418 C>T Type: Missense REVEL: 0.12 (Mod Benign) | VUS | |
12 | TBD | TERC | n.116C>G | Non-coding | TL: < 1%ile (FF) | PM2 PS3 | NR_001566.1 chr3-169482733 G>C | LPV | |
13 | B-ALL | IKZF3 | c.244G>A | p.Glu82Lys | SNV | PM2 BP4 | NM_012481.5 chr17-37949106 C>T Type: Missense REVEL: 0.09 (Mod Benign) | VUS | |
14 | AML | TINF2 | c.100G>C | p.Val34Leu | Not done | SNV | PM2 | NM_001099274.3 chr14-24711439 C>G Type: Missense REVEL: 0.3 (Uncertain) | VUS |
15 | HBOC | BRCA1 | c.1874_1877dup | p.Val627fs*4 | Dup | PS4 PVS1 PM2 | NM_007294.4 chr17-41245670 T>TACTA Type: Frameshift indel | PV | |
TBD | RTEL1 | c.897del | p.Phe299Leufs*10 | Pending | Del | PVS1 PM2 PP5 | NM_001283009.2 chr20-62305423 TC>T Type: Frameshift indel | PV | |
TNFRSF13B | c.542C>A | p.Ala181Glu | SNV | PM2 PP5 | NM_012452.3 chr17-16843729 G>T Type: Missense REVEL: 0.61 (Uncertain) | LPV | |||
16 | HBOC | BRCA1 | c.1874_1877dup | p.Val627fs*4 | Dup | PS4 SNVPVS1 PM2 | NM_007294.4 chr17-41245670 T>TACTA Type: Frameshift indel | PV | |
TBD | RTEL1 | c.897del | p.Phe299Leufs*10 | Normal | Del | PVS1 PM2 PP5 | NM_001283009.2 chr20-62305423 TC>T Type: Missense | PV | |
TNFRSF13B | c.542C>A | p.Ala181Glu | SNV | PM2 PP5 | NM_007294.4 chr17-41245670 T>TACTA Type: Missense | PV |
We found that patients with shortened telomeres had delta telomere lengths for lymphocytes and granulocytes less than -2.00. We also summarized these results in eight patients within our cohort and the relevant genes associated with TBD in Figure 2.
Clinical outcomes were significant for two deaths during this period, leading to a mortality rate of 12.5%. One was due to respiratory failure because of pulmonary fibrosis secondary to an underlying diagnosis of TBD. Another was due to relapsed acute myeloid leukemia. A full summary of the clinical phenotypes and referral indications are in the Supplementary Table 1.
DISCUSSION
Overall, the diagnostic yield of clinical-grade genomic testing in a selected population of patients referred for suspected IBMFS is approximately 50%. Although one of the limitations of this study is the small number of patients (n = 16), it is interesting to note that approximately 25% of patients may have dual diagnoses that were not apparent prior to genetic testing. We also identified patients at an earlier age through increased access to exome sequencing in patients presenting with multiple congenital anomalies, global developmental delays, and intellectual disability based on a recent ACMG guideline published in 2021[51]. We also acknowledge the potential referral bias in this cohort as these patients were referred by other healthcare providers based on the presence of cytopenias concerning an underlying IBMFS, personal and/or family history of a hematological malignancy, solid tumor or extra-hematopoietic organ dysfunction associated with IBMFS, personal history of pre-existing cytopenia(s), and abnormal genetic test results in genes related to IBMFS. The lack of a standardized NGS panel with a fixed number of genes tested reflects this heterogeneity in this patient population and highlights the complexities in clinical care when patients receive genetic testing through other healthcare providers.
Some of the challenges encountered in the clinical setting included difficulty in obtaining a skin punch biopsy for patients who presented with acute myeloid leukemia (AML) and myelodysplastic syndrome (MDS) at diagnosis. Given the presence of somatic variants and the possibility of clonal hematopoiesis in peripheral blood, it is recommended that germline genetic testing be performed on non-hematopoietic tissue, such as cultured skin fibroblast[52]. We overcame this challenge by performing the skin punch biopsy during the repeat bone marrow biopsy after induction treatment. For patients who have undergone a prior HSCT, skin punch biopsy was performed by the clinical geneticist in the outpatient clinic. In an ideal situation, this procedure could be performed when the diagnostic bone marrow biopsy is scheduled, especially in a young patient with a suspected germline predisposition to myeloid neoplasms. Other tissue sources such as eyebrow hair follicles, fingernail clippings, and buccal swabs have been evaluated as possible alternative sources of germline DNA in patients with MDS[53] or AML[54]. A recent practice resource by the National Society of Genetic Counselors has highlighted the caveats and potential pitfalls such as the risk of clonal hematopoiesis of indeterminate potential (CHIP) when using other sample sources like blood, saliva, or buccal swabs for germline genetic testing in patients with hematological malignancies[55].
Another challenge encountered is the inability to send ancillary tests that would help clarify any VUS that is reported from genomic testing. One of the reasons that these tests were not able to be sent (e.g., telomere length or chromosomal breakage analysis) was leukopenia during active disease or because of active chemotherapy. Culture failure of skin fibroblasts was a rare event that occurred only in one patient (11%).
In addition to the challenges mentioned above, the VUS rate is approximately 50%, with eight patients having at least one VUS detected in their genomic test. Functional studies are required to clarify the pathogenicity of these VUS but are not always available, and the development of in vitro assays requires significant investment in terms of time, personnel, and funding. Novel methods to improve in silico algorithms that predict pathogenicity may include saturation mutagenesis-reinforced functional assays (SMuRF)[56]. Innovative methods for telomere length measurement, which can be performed on non-hematopoietic tissues, including in patients post-HSCT, would be highly desirable and useful in the clinical setting, and some research groups have attempted to address this challenge using long-read sequencing technology[57-59]. The use of multi-omics[60,61] such as transcriptomics[62], metabolomics[63], and proteomics[64], as well as novel disease models[65] using induced pluripotent stem cells (iPSCs) or organoids[66,67], have been used to clarify VUS in patients with undiagnosed diseases and should be used in the analysis of the remaining 50% of these IBMFS patients that remain genomically uncharacterized.
For patients with dual diagnoses, further studies have been planned to utilize multi-omics and novel cellular-based disease models to identify the pathomechanisms and interacting pathways in these patients with two rare disorders.
Finally, some patients were diagnosed genomically but did not exhibit the typical clinical phenotype associated with bone marrow failure given their young age. Early diagnosis in younger individuals is important to better understand these rare disorders and to direct screening and surveillance plans. Continued long-term follow-up is very important to understand the natural history of patients with these underlying genetic variants associated with IBMFS and their risk of solid and hematological malignancies.
CONCLUSION
Early diagnosis of an underlying IBMFS is important to reduce mortality and morbidity. While there is increased awareness among providers of the multi-organ involvement in IBMFS and the risk of malignancies, access to genetic evaluation and counseling may be limited or delayed in certain circumstances. We highlighted our institutional experience within the Division of Medical Genetics and Genomics and the challenges involved in the diagnosis and management of these patients who are suspected of having an underlying IBMFS. In this selected group of patients, we identified a genomic diagnosis in half of the patients while the remaining patients were genomically uncharacterized. Ancillary tests such as erythrocyte adenosine deaminase, telomere lengths, and chromosomal breakage analysis are very useful in clarifying variants of uncertain significance and should be performed early during the clinical evaluation of these patients. Although overall mortality is improving with better supportive care, curative options are still limited. Continued research is required in this field to better understand disease-causing coding and non-coding variants in patients with IBMFS - clarifying the pathogenicity of variants of uncertain significance, consequences of gene interactions in patients with dual diagnoses, and the underlying mechanisms of disease in this group of patients. Natural history studies, in combination with translational research, are required to improve the mortality and morbidity in these patients.
DECLARATIONS
Authors’ contributions
Involved in the clinical care, enrollment of the patients in the study, coordinating/performing skin punch biopsy, ancillary and genetic testing, and writing of the manuscript: Dillahunt K, Younger G, Van Der Graaf M, Tung ML
Involved in the conceptualization of the study, analysis of the data and editing of the manuscript: Chandra B, Tung ML
Availability of data and materials
The data are available from the corresponding author upon reasonable request.
Financial support and sponsorship
This work was supported by the University of Iowa Stead Family Department of Pediatrics Research Grant Program.
Conflicts of interest
Chandra B, Tung ML are Youth Editorial Board members of Journal of Translational Genetics and Genomics. Chandra B, Tung ML were not involved in any steps of editorial processing, notably including reviewer selection, manuscript handling, and decision making, while the other authors have declared that they have no conflicts of interest.
Ethical approval and consent to participate
A written informed consent for publication was obtained.
Consent for publication
Not applicable.
Copyright
© The Author(s) 2025.
Supplementary Materials
REFERENCES
1. Germeshausen M, Ballmaier M. Congenital amegakaryocytic thrombocytopenia - not a single disease. Best Pract Res Clin Haematol. 2021;34:101286.
3. Dobrewa W, Bielska M, Bąbol-Pokora K, Janczar S, Młynarski W. Congenital neutropenia: from lab bench to clinic bedside and back. Mutat Res Rev Mutat Res. 2024;793:108476.
4. Revy P, Kannengiesser C, Bertuch AA. Genetics of human telomere biology disorders. Nat Rev Genet. 2023;24:86-108.
5. Tummala H, Walne AJ, Badat M, et al. The evolving genetic landscape of telomere biology disorder dyskeratosis congenita. EMBO Mol Med. 2024;16:2560-82.
6. Boussion S, Escande F, Jourdain AS, et al. TAR syndrome: clinical and molecular characterization of a cohort of 26 patients and description of novel noncoding variants of RBM8A. Hum Mutat. 2020;41:1220-5.
7. Da Costa L, Mohandas N, David-NGuyen L, et al. Diamond-blackfan anemia, the archetype of ribosomopathy: how distinct is it from the other constitutional ribosomopathies? Blood Cells Mol Dis. 2024;106:102838.
8. Kawashima N, Oyarbide U, Cipolli M, Bezzerri V, Corey SJ. Shwachman-Diamond syndromes: clinical, genetic, and biochemical insights from the rare variants. Haematologica. 2023;108:2594-605.
9. Bhandari J, Thada PK, Killeen RB, Puckett Y. Fanconi anemia. Treasure Island: StatPearls Publishing; 2024.
11. Lee JM, Kim HS, Yoo J, et al. Genomic insights into inherited bone marrow failure syndromes in a Korean population. Br J Haematol. 2024;205:1581-9.
12. Gutierrez-Rodrigues F, Patel BA, Groarke EM. When to consider inherited marrow failure syndromes in adults. Hematol Am Soc Hematol Educ Program. 2023;2023:548-55.
13. Fox LC, McQuilten ZK, Firkin F, et al. The Australian aplastic Anaemia and other bone marrow failure syndromes registry. Best Pract Res Clin Haematol. 2023;36:101516.
14. Leal-Anaya P, Kimball TN, Yanez-Felix AL, et al. Inherited bone marrow failure syndromes: phenotype as a tool for early diagnostic suspicion at a major reference center in Mexico. Front Genet. 2023;14:1293929.
16. Deng J, McReynolds LJ. Inherited bone marrow failure syndromes: a review of current practices and potential future research directions. Curr Opin Pediatr. 2023;35:75-83.
17. Groarke EM, Calado RT, Liu JM. Cell senescence and malignant transformation in the inherited bone marrow failure syndromes: overlapping pathophysiology with therapeutic implications. Semin Hematol. 2022;59:30-7.
18. Lipton JM, Molmenti CLS, Desai P, Lipton A, Ellis SR, Vlachos A. Early onset colorectal cancer: an emerging cancer risk in patients with diamond Blackfan anemia. Genes. 2021;13:56.
19. Schratz KE, Haley L, Danoff SK, et al. Cancer spectrum and outcomes in the Mendelian short telomere syndromes. Blood. 2020;135:1946-56.
20. Alter BP, Giri N, Savage SA, Rosenberg PS. Cancer in the national cancer institute inherited bone marrow failure syndrome cohort after fifteen years of follow-up. Haematologica. 2018;103:30-9.
21. Keel SB, Scott A, Sanchez-Bonilla M, et al. Genetic features of myelodysplastic syndrome and aplastic anemia in pediatric and young adult patients. Haematologica. 2016;101:1343-50.
22. Gálvez E, Vallespín E, Arias-Salgado EG, et al. Next-generation sequencing in bone marrow failure syndromes and isolated cytopenias: experience of the spanish network on bone marrow failure syndromes. Hemasphere. 2021;5:e539.
23. Ghemlas I, Li H, Zlateska B, et al. Improving diagnostic precision, care and syndrome definitions using comprehensive next-generation sequencing for the inherited bone marrow failure syndromes. J Med Genet. 2015;52:575-84.
24. Zhang MY, Keel SB, Walsh T, et al. Genomic analysis of bone marrow failure and myelodysplastic syndromes reveals phenotypic and diagnostic complexity. Haematologica. 2015;100:42-8.
25. Muramatsu H, Okuno Y, Yoshida K, et al. Clinical utility of next-generation sequencing for inherited bone marrow failure syndromes. Genet Med. 2017;19:796-802.
26. Bluteau O, Sebert M, Leblanc T, et al. A landscape of germ line mutations in a cohort of inherited bone marrow failure patients. Blood. 2018;131:717-32.
27. Blombery P, Fox L, Ryland GL, et al. Utility of clinical comprehensive genomic characterization for diagnostic categorization in patients presenting with hypocellular bone marrow failure syndromes. Haematologica. 2021;106:64-73.
28. Goodwin S, McPherson JD, McCombie WR. Coming of age: ten years of next-generation sequencing technologies. Nat Rev Genet. 2016;17:333-51.
29. Kulasekararaj A, Cavenagh J, Dokal I, et al. Guidelines for the diagnosis and management of adult aplastic anaemia: a British society for haematology guideline. Br J Haematol. 2024;204:784-804.
30. Shimano KA, Narla A, Rose MJ, et al. Diagnostic work-up for severe aplastic anemia in children: consensus of the North American pediatric aplastic anemia consortium. Am J Hematol. 2021;96:1491-504.
31. Roy NBA, Da Costa L, Russo R, et al. The use of next-generation sequencing in the diagnosis of rare inherited anaemias: a joint BSH/EHA good practice paper. Hemasphere. 2022;6:e739.
32. Khoury JD, Solary E, Abla O, et al. The 5th edition of the World Health Organization classification of haematolymphoid tumours: myeloid and histiocytic/dendritic neoplasms. Leukemia. 2022;36:1703-19.
33. Döhner H, Wei AH, Appelbaum FR, et al. Diagnosis and management of AML in adults: 2022 recommendations from an international expert panel on behalf of the ELN. Blood. 2022;140:1345-77.
34. Arber DA, Orazi A, Hasserjian RP, et al. International consensus classification of myeloid neoplasms and acute leukemia: integrating morphologic, clinical, and genomic data. Blood. 2022;140:1200-28.
35. Greenberg PL, Stone RM, Al-Kali A, et al. NCCN guidelines® insights: myelodysplastic syndromes, version 3.2022. J Natl Compr Canc Netw. 2022;20:106-17.
36. Feurstein S, Hahn CN, Mehta N, Godley LA. A practical guide to interpreting germline variants that drive hematopoietic malignancies, bone marrow failure, and chronic cytopenias. Genet Med. 2022;24:931-54.
37. Gutierrez-Rodrigues F, Sahoo SS, Wlodarski MW, Young NS. Somatic mosaicism in inherited bone marrow failure syndromes. Best Pract Res Clin Haematol. 2021;34:101279.
38. Schratz KE, Gaysinskaya V, Cosner ZL, et al. Somatic reversion impacts myelodysplastic syndromes and acute myeloid leukemia evolution in the short telomere disorders. J Clin Invest. 2021;131:e147598.
39. Trottier AM, Godley LA. Inherited predisposition to haematopoietic malignancies: overcoming barriers and exploring opportunities. Br J Haematol. 2021;194:663-76.
40. McReynolds LJ, Rafati M, Wang Y, et al. Genetic testing in severe aplastic anemia is required for optimal hematopoietic cell transplant outcomes. Blood. 2022;140:909-21.
41. Agarwal S. Minimal intensity conditioning strategies for bone marrow failure: is it time for "preventative" transplants? Hematol Am Soc Hematol Educ Program. 2023;2023:135-40.
42. Alter BP. Inherited bone marrow failure syndromes: considerations pre- and posttransplant. Hematol Am Soc Hematol Educ Program. 2017;2017:88-95.
43. Atmar K, Ruivenkamp CAL, Hooimeijer L, et al. Diagnostic value of a protocolized in-depth evaluation of pediatric bone marrow failure: a multi-center prospective cohort study. Front Immunol. 2022;13:883826.
44. Bonfim C. Special pre- and posttransplant considerations in inherited bone marrow failure and hematopoietic malignancy predisposition syndromes. Hematol Am Soc Hematol Educ Program. 2020;2020:107-14.
45. Dietz AC, Mehta PA, Vlachos A, et al. Current knowledge and priorities for future research in late effects after hematopoietic cell transplantation for inherited bone marrow failure syndromes: consensus statement from the second pediatric blood and marrow transplant consortium international conference on late effects after pediatric hematopoietic cell transplantation. Biol Blood Marrow Transplant. 2017;23:726-35.
46. Dietz AC, Savage SA, Vlachos A, et al. Late effects screening guidelines after hematopoietic cell transplantation for inherited bone marrow failure syndromes: consensus statement from the second pediatric blood and marrow transplant consortium international conference on late effects after pediatric HCT. Biol Blood Marrow Transplant. 2017;23:1422-8.
47. Hudda Z, Myers KC. Posttransplant complications in patients with marrow failure syndromes: are we improving long-term outcomes? Hematol Am Soc Hematol Educ Program. 2023;2023:141-8.
48. Richards S, Aziz N, Bale S, et al. Standards and guidelines for the interpretation of sequence variants: a joint consensus recommendation of the American College of Medical Genetics and Genomics and the Association for Molecular Pathology. Genet Med. 2015;17:405-24.
49. Riggs ER, Andersen EF, Cherry AM, et al. Technical standards for the interpretation and reporting of constitutional copy-number variants: a joint consensus recommendation of the American College of Medical Genetics and Genomics (ACMG) and the Clinical Genome Resource (ClinGen). Genet Med. 2020;22:245-57.
50. Alder JK, Hanumanthu VS, Strong MA, et al. Diagnostic utility of telomere length testing in a hospital-based setting. Proc Natl Acad Sci USA. 2018;115:E2358-65.
51. Manickam K, McClain MR, Demmer LA, et al. Exome and genome sequencing for pediatric patients with congenital anomalies or intellectual disability: an evidence-based clinical guideline of the American College of Medical Genetics and Genomics (ACMG). Genet Med. 2021;23:2029-37.
52. Godley LA, DiNardo CD, Bolton K. Germline predisposition in hematologic malignancies: testing, management, and implications. Am Soc Clin Oncol Educ Book. 2024;44:e432218.
53. Padron E, Ball MC, Teer JK, et al. Germ line tissues for optimal detection of somatic variants in myelodysplastic syndromes. Blood. 2018;131:2402-5.
54. Ceyhan-Birsoy O, Fiala E, Rana S, et al. Universal germline genetic testing in patients with hematologic malignancies using DNA isolated from nail clippings. Haematologica. 2024;109:3383-90.
55. Stewart BL, Helber H, Bannon SA, et al. Risk assessment and genetic counseling for hematologic malignancies-Practice resource of the National Society of Genetic Counselors. J Genet Couns. 2025;34:e1959.
56. Ma K, Huang S, Ng KK, et al. Saturation mutagenesis-reinforced functional assays for disease-related genes. Cell. 2024;187:6707-24.e22.
57. Sanchez SE, Gu Y, Wang Y, et al. Digital telomere measurement by long-read sequencing distinguishes healthy aging from disease. Nat Commun. 2024;15:5148.
58. Tham CY, Poon L, Yan T, et al. High-throughput telomere length measurement at nucleotide resolution using the PacBio high fidelity sequencing platform. Nat Commun. 2023;14:281.
59. Sholes SL, Karimian K, Gershman A, Kelly TJ, Timp W, Greider CW. Chromosome-specific telomere lengths and the minimal functional telomere revealed by nanopore sequencing. Genome Res. 2022;32:616-28.
60. Smirnov D, Konstantinovskiy N, Prokisch H. Integrative omics approaches to advance rare disease diagnostics. J Inherit Metab Dis. 2023;46:824-38.
61. Marwaha S, Knowles JW, Ashley EA. A guide for the diagnosis of rare and undiagnosed disease: beyond the exome. Genome Med. 2022;14:23.
62. Montgomery SB, Bernstein JA, Wheeler MT. Toward transcriptomics as a primary tool for rare disease investigation. Cold Spring Harb Mol Case Stud. 2022;8:a006198.
63. Cummings BB, Marshall JL, Tukiainen T, et al. Improving genetic diagnosis in Mendelian disease with transcriptome sequencing. Sci Transl Med. 2017;9:eaal5209.
64. Zech M, Dzinovic I, Skorvanek M, et al. Combined genomics and proteomics unveils elusive variants and vast aetiologic heterogeneity in dystonia. Brain. 2025:awaf059.
65. Musunuru K, Bernstein D, Cole FS, et al. Functional assays to screen and dissect genomic hits: doubling down on the national investment in genomic research. Circ Genom Precis Med. 2018;11:e002178.
66. Olijnik AA, Rodriguez-Romera A, Wong ZC, et al. Generating human bone marrow organoids for disease modeling and drug discovery. Nat Protoc. 2024;19:2117-46.
Cite This Article

How to Cite
Download Citation
Export Citation File:
Type of Import
Tips on Downloading Citation
Citation Manager File Format
Type of Import
Direct Import: When the Direct Import option is selected (the default state), a dialogue box will give you the option to Save or Open the downloaded citation data. Choosing Open will either launch your citation manager or give you a choice of applications with which to use the metadata. The Save option saves the file locally for later use.
Indirect Import: When the Indirect Import option is selected, the metadata is displayed and may be copied and pasted as needed.
About This Article
Copyright
Data & Comments
Data
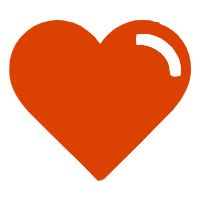
Comments
Comments must be written in English. Spam, offensive content, impersonation, and private information will not be permitted. If any comment is reported and identified as inappropriate content by OAE staff, the comment will be removed without notice. If you have any queries or need any help, please contact us at support@oaepublish.com.