Genetic study of the NUS1 gene variants in Han Chinese patients with Parkinson’s disease
Abstract
Aim: Parkinson’s disease (PD) is the second most common progressive neurodegenerative disease linked to genetic and other factors. The NUS1 dehydrodolichyl diphosphate synthase subunit gene (NUS1) variants were reported to be associated with PD. In this PD-control cohort, we aimed to explore the potential role of the NUS1 gene variants in PD.
Methods: A cohort of 512 Han Chinese sporadic PD patients and 516 ethnically and age-matched controls underwent clinical evaluation. Peripheral blood samples were then collected, and whole-exome sequencing was performed. The potential PD-related variants identified through screening were verified using Sanger sequencing, further classified, and subsequently analyzed by bioinformatics analysis tools. Statistical analysis was conducted to assess the association between the variants and PD.
Results: Three NUS1 heterozygous missense variants, including c.127G>T (p.Ala43Ser, rs1327892878), c.487G>C (p.Asp163His, rs369403261), and c.537T>A (p.Asp179Glu, rs28362519), were identified. Two rare variants, c.127G>T and c.487G>C, were exclusively found in PD patients, while the low-frequency variant c.537T>A was detected both in patients and controls. Combined with bioinformatics analysis, a potentially pathogenic role of c.127G>T and c.487G>C may exert in PD risk, though no significant association was shown by statistical analysis (all P > 0.05).
Conclusion: Our findings suggested that the NUS1 variants seem to not cause monogenic PD, and variants like c.127G>T and c.487G>C may, at most, exert a susceptibility to PD.
Keywords
INTRODUCTION
Parkinson’s disease (PD) is the second most prevalent progressive neurodegenerative condition after Alzheimer’s disease[1,2]. The globally pooled all-age prevalence of PD is 0.151%, which has been on the rise, especially over the past two decades, with the prevalence higher in males (0.154%) than females (0.149%), increasing with age[3]. According to the Movement Disorder Society Clinical Diagnostic Criteria for PD (MDS-PD Criteria) in 2015, it centers on motor syndrome, including the cardinal manifestations of bradykinesia, rest tremor, and rigidity[4]. This heterogeneous neurological disorder, which is pathologically characterized by aberrant deposition of α-synuclein and death of dopaminergic neurons in the substantia nigra, as well as nondopaminergic changes, presents various motor and non-motor symptoms[5-7]. It is tied to a complex interplay of factors including genetics, aging, and environmental risk[8,9], among which genetic factors are responsible for ~30% of PD cases, with 5%-10% following a monogenic inheritance pattern[9,10-12].
Genome-wide association studies (GWAS) have provided new insights into the pathophysiology of PD, as over 90 independent significant risk variants have been revealed to be correlated with PD[13,14], and
The NUS1 dehydrodolichyl diphosphate synthase subunit gene (NUS1) was first identified as a candidate gene for PD through trio or quad whole-exome sequencing (WES), independent case-control analysis, and Drosophila study[19]. Several subsequent studies have revealed rare or low-frequency NUS1 gene variants contributing to PD or have reported controversial conclusions[20-25], indicating the veiled effects of the NUS1 gene variants in PD.
In this study, we aimed to screen the NUS1 gene variants in a PD-control cohort and evaluate the potential impacts on PD in the Han Chinese population, which may provide new insights into the NUS1-related genetic contribution to PD etiology.
METHODS
Study participants and clinical evaluations
A total of 512 unrelated Han Chinese sporadic PD patients (male/female: 263/249, mean age at sampling: 59.36 ± 10.92 years, mean age at onset: 55.93 ± 10.15 years) and 516 ethnically and age-matched controls (male/female: 263/253, mean age at sampling: 60.11 ± 9.76 years) without PD-related manifestations or family history were enrolled in our cohort, of which 216 sporadic PD patients and 308 controls previously evaluated were included[23]. According to the MDS-PD Criteria, the patients were diagnosed by two proficient neurologists. The related clinical information and peripheral blood samples were taken from all participants after obtaining written informed consent. This study was approved by the Institutional Review Board of the Third Xiangya Hospital, Central South University, Changsha, Hunan, China. All procedures followed the ethical guidelines of the Declaration of Helsinki due to human samples involved.
DNA extraction and whole-exome sequencing
Genomic DNA (gDNA) was isolated from peripheral blood using the previously reported standard
Variant analysis
The raw data were filtered to obtain clean reads for subsequent bioinformatics analysis. The
Bioinformatics analysis
The pathogenicity of potential variants was assessed using the bioinformatics prediction tools, Combined Annotation Dependent Depletion (CADD), Polymorphism Phenotyping v2 (PolyPhen-2), Sorting Intolerant from Tolerant (SIFT), Protein Variation Effect Analyzer (PROVEAN), MutPred2, Functional Analysis through Hidden Markov Models (FATHMM), and MutationTaster2021[18,26,33]. The protein stability changes related to substitution variants were predicted by the sequence-based Single Amino Acid Folding free Energy Changes (SAAFEC-SEQ) (http://compbio.clemson.edu/SAAFEC-SEQ/) and machine-learning MUpro (http://mupro.proteomics.ics.uci.edu/) online tools[34,35]. The NCBI Basic Local Alignment Search Tool (BLAST, https://blast.ncbi.nlm.nih.gov/Blast.cgi) was used to evaluate the sequence conservation of amino acids at variant positions among 10 species.
Statistical analysis
Pearson’s chi-square test was performed to assess the gender difference in cases and controls, and an independent samples t-test was used to assess the age difference. Deviation from Hardy-Weinberg equilibrium was tested in controls. Pearson’s chi-square test or Fisher’s exact test was applied to test genotypic and allelic frequency differences between case and control groups. The statistical analysis was performed using Statistical Product and Service Solutions (version 24, SPSS Inc., Chicago, IL, USA). The P value < 0.05 on both sides was considered statistically significant. The gene-based analysis, optimized sequence kernel association test (SKAT-O) in R-4.4.1, was applied to analyze the obtained rare variants in both groups, with gender and age at sampling as covariates.
RESULTS
A total of three NUS1 (NM_138459.5) heterozygous missense variants, including c.127G>T (p.Ala43Ser, rs1327892878), c.487G>C (p.Asp163His, rs369403261), and c.537T>A (p.Asp179Glu, rs28362519), were revealed by WES and confirmed by Sanger sequencing in the cohort [Figure 1A]. All three variants were detected in sporadic PD cases, with one also identified in controls. The p.Ala43Ser variant was found in two PD patients with onset age over 50 years, and the p.Asp163His variant was discovered in a 73-year-old female patient suffering from bradykinesia, rigidity, and postural instability [Table 1]. The p.Asp179Glu variant was detected in 7 PD patients and 9 controls. Two variants p.Ala43Ser and p.Asp163His with a
Figure 1. The three identified NUS1 heterozygous missense variants, c.127G>T (p.Ala43Ser), c.487G>C (p.Asp163His), and c.537T>A (p.Asp179Glu). (A) The Sanger sequencing results of three missense variants, as well as a synonymous variant (c.126C>A, p.Arg42=, rs947563301) co-occurring with c.127G>T; (B) Sequence alignment of the NUS1-encoded NgBR protein in different species, with arrows pointing to the amino acids affected by the variants; (C) The locations of three variants in the pattern diagram and topological model of NgBR protein, mainly localized to the endoplasmic reticulum. NUS1: the NUS1 dehydrodolichyl diphosphate synthase subunit gene; TM: transmembrane domain; aa: amino acid; cis-PTase homology: cis-prenyltransferase homology domain; ER: endoplasmic reticulum; NgBR: neurite outgrowth inhibitor B receptor.
Clinical data of three PD patients with the NUS1 c.127G>T (p.Ala43Ser) and c.487G>C (p.Asp163His) variants
Item | Case 1 | Case 2 | Case 3 |
NUS1 variant | c.127G>T (p.Ala43Ser) | c.127G>T (p.Ala43Ser) | c.487G>C (p.Asp163His) |
Gender | Male | Female | Female |
Age at onset | 52 years old | 71 years old | 70 years old |
Age at sampling | 54 years old | 73 years old | 73 years old |
Family history | No | No | No |
Symptoms at onset | Involuntary shaking of hands | Progressive bradykinesia and involuntary shaking of the right limb and jaw | Bradykinesia and postural deformity |
Motor features | |||
Bradykinesia | No | Yes | Yes |
Rest tremor (distribution) | Yes (two hands) | Yes (right limb) | No |
Rigidity | Yes | No | Yes |
Muscle tone | Increased | Increased | Increased |
Muscle strength | Normal | Normal | Normal |
Gait disturbance | No | Yes (festination) | Yes (festination) |
Imbalance/impaired postural reflexes | No | No | Yes |
Dysarthria | No | No | No |
Treatment (levodopa) | 375 mg/day | 187.5 mg/day | 562.5 mg/day |
Response to levodopa | Poor | Good | Good |
Levodopa-induced dyskinesia | No | No | No |
Sensory abnormalities | No | No | Yes |
Reflex | |||
Plantar response | Normal | Normal | Normal |
Babinski sign | No | No | No |
Cognitive decline | No | No | No |
Psychiatric features | No | No | No |
Sleeping dysfunction | Yes | Yes | Yes |
Autonomic involvement | No | Yes (constipation) | No |
Hyposmia | No | Yes | No |
Others | |||
Cerebellar signs | No | No | No |
Seizures | No | No | No |
Brain magnetic resonance imaging | Scattered ischemic foci in frontal and parietal lobes | A few ischemic foci in white matter | Scattered brain ischemic foci |
The variants, p.Ala43Ser and p.Asp163His, were predicted to be conflicting, in which over half of the pathogenicity prediction tools (4/7) predicted p.Asp163His as pathogenic, while all the tools predicted p.Asp179Glu as benign. Two missense variants, p.Ala43Ser and p.Asp163His, were predicted to reduce the protein stability by SAAFEC-SEQ or MUpro, while p.Asp179Glu was predicted to reduce the stability only by MUpro [Supplementary Table 1]. The amino acid residues, p.Ala43, p.Asp163, and p.Asp179, are highly conserved in the nine species from reptiles to mammals, except in lower organisms below zebrafish [Figure 1B].
No statistically significant difference was found in terms of gender or age between patients and controls (both P > 0.05). The Hardy-Weinberg equilibrium test indicated no deviation in the controls (all P > 0.05). Absent in controls, two potential PD-associated variants, p.Ala43Ser and p.Asp163His, had the genotype frequency of 0.39% and 0.20% in the case population, respectively. No statistically significant differences in genotypic distributions or allele frequencies between 512 PD patients and 516 controls (all P > 0.05) were revealed in three variants [Supplementary Table 2]. Further gene-based SKAT-O analysis showed no significant association between the identified rare variant and the PD phenotype (P = 0.1494 for p.Ala43Ser and P = 0.2893 for p.Asp163His), even combined (P = 0.1259 for two rare variants p.Ala43Ser and p.Asp163His, and P = 0.5784 for all three variants).
DISCUSSION
PD is a common complex neurodegenerative disease determined by monogenic variants or related to multiple factors[36,37]. Since the watershed in 1997, when a pathogenic variant of the SNCA gene, known as the first PD-causative gene, was identified in autosomal dominant PD families[38], a large number of genetic loci have been implicated in PD[39]. To date, at least 26 loci and 22 genes have been definitively identified as responsible for monogenic PD[39-41], although they account for less than 10% of cases[42,43]. Additionally, variants in many susceptibility genes have been reported to increase the risk of PD development, recognized as common risk factors[44-46]. In recent years, a research group has reported that the NUS1 gene variants are associated with PD mainly in Chinese[19-21]. In contrast, other studies have suggested a lack of association between the NUS1 gene variants and PD in case-control cohorts of Chinese or European descent[23-25].
The NUS1 gene, located on chromosome 6q22.1, contains 5 exons and encodes the transmembrane protein, neurite outgrowth inhibitor B (Nogo-B) receptor (NgBR), which is a subunit of cis-prenyltransferase
In this study, we identified three NUS1 heterozygous missense variants in our cohort, of which two variants, c.127G>T (p.Ala43Ser) and c.487G>C (p.Asp163His), were found only in patients, and
However, we suspected that p.Asp163His and p.Ala43Ser may pose a risk for PD in the population, which is supported by the following evidence: (i) Some de novo heterozygous variants in the NUS1 gene were disease-causing variants for MRD55[55]. (ii) To date, no co-segregated disease-causing NUS1 variant has been found in a large family with PD[19]. (iii) These two variants had low frequencies in the population and their bioinformatics predictions were conflicting, especially for the p.Ala43Ser variant. (iv) These two variants were identified in sporadic PD cases rather than familial cases. (v) The amino acids are highly conserved in multi-species. (vi) Particularly, the TM2 domain that the p.Ala43Ser affects may not be a determiner in the orientation of the C-terminal like TM1 and TM3 [Figure 1C]. Burden analysis of rare variants including p.Asp163His was reported to be deleterious and have an association with PD[19-21]. Although statistical analysis showed no associations between the variants (p.Ala43Ser and p.Asp163His) and PD phenotype, combined with all the findings, we could not fully exclude a possible role in PD. Moreover, PD is a typical age-related neurological disease. These three patients were all over 50 years at age onset and had no positive family history, which suggested that the NUS1 gene variants may play a role in increasing susceptibility in PD at most, rather than cause PD in a monogenic inheritance pattern with low penetrance.
Furthermore, even though p.Ala43Ser and p.Asp163His may potentially contribute to PD susceptibility, the NUS1 variant(s) may affect less than 0.6% (3/512) of Han Chinese patients with PD. More studies with large-scale samples, as well as high-throughput sequencing approaches and valid bioinformatics tools, are required to verify the role of NUS1 gene variants in PD susceptibility. In conjunction with further screening of cases with neurological disorders, including CDG1AA and MRD55, elucidating the role of the NUS1 gene in neurological diseases, particularly neurodevelopmental conditions, will become touchable.
In summary, our study indicated that the NUS1 gene variants seem to not cause monogenic PD, which may exert a susceptibility to PD at most. The findings enhanced the understanding of the real impact of the NUS1 gene variants on monogenic PD by the limited samples in this study.
DECLARATIONS
Acknowledgments
The authors sincerely thank all participants and researchers for their support and cooperation in collecting DNA samples, clinical data, and genetic information.
Authors’ contributions
Research conception and design: Gao C, Yuan L, Zheng W, Deng H
Data acquisition and analysis: Gao C, Yuan L, Zheng W, Yang Y, Song Z, Guo Y, Deng H
Writing & original draft: Gao C, Yuan L, Deng H
Writing & review and editing: Gao C, Yuan L, Deng H
Availability of data and materials
The data supporting the findings of this study are available within this Article and its [Supplementary Materials]. All data obtained during the study are available from the corresponding author upon reasonable request.
Financial support and sponsorship
This work was supported by National Natural Science Foundation of China (No. 81873686), Natural Science Foundation of Hunan Province (No. 2023JJ30715), Scientific Key Research Project of Health Commission of Hunan Province (No. A202303018385), Health Research Project of Hunan Provincial Health Commission (No. W20243024), Natural Science Foundation of Changsha (No. kq2403186), Distinguished Professor of the Lotus Scholars Award Program of Hunan Province, Sublimation Scholars Project of Central South University, and Wisdom Accumulation and Talent Cultivation Project of the Third Xiangya Hospital of Central South University (No. YX202109).
Conflicts of interest
Deng H is an Editorial Board member of the journal Ageing and Neurodegenerative Diseases. Deng H was not involved in any steps of editorial processing, notably including reviewer selection, manuscript handling, and decision making. The other authors declared that there are no conflicts of interest.
Ethical approval and consent to participate
The study involving human subjects was conducted in strict accordance with the Declaration of Helsinki and approved by the Institutional Review Board of the Third Xiangya Hospital, Central South University, Changsha, Hunan, China (No. 2018-S400). Clinical data and peripheral blood samples were collected from patients after obtaining written informed consent.
Consent for publication
Not applicable.
Copyright
© The Author(s) 2025.
Supplementary Materials
REFERENCES
1. Chhetri JK, Mei S, Wang C, Chan P. New horizons in Parkinson's disease in older populations. Age Ageing. 2023;52:afad186.
2. Deliz JR, Tanner CM, Gonzalez-Latapi P. Epidemiology of Parkinson's disease: an update. Curr Neurol Neurosci Rep. 2024;24:163-79.
3. Zhu J, Cui Y, Zhang J, et al. Temporal trends in the prevalence of Parkinson's disease from 1980 to 2023: a systematic review and meta-analysis. Lancet Healthy Longev. 2024;5:e464-79.
4. Postuma RB, Berg D, Stern M, et al. MDS clinical diagnostic criteria for Parkinson's disease. Mov Disord. 2015;30:1591-601.
5. Geng L, Gao W, Saiyin H, et al. MLKL deficiency alleviates neuroinflammation and motor deficits in the α-synuclein transgenic mouse model of Parkinson's disease. Mol Neurodegener. 2023;18:94.
6. Muwanigwa MN, Modamio-Chamarro J, Antony PMA, et al. Alpha-synuclein pathology is associated with astrocyte senescence in a midbrain organoid model of familial Parkinson's disease. Mol Cell Neurosci. 2024;128:103919.
7. González-Casacuberta I, Vilas D, Pont-Sunyer C, et al. Neuronal induction and bioenergetics characterization of human forearm adipose stem cells from Parkinson's disease patients and healthy controls. PLoS One. 2022;17:e0265256.
8. Lim SY, Klein C. Parkinson's disease is predominantly a genetic disease. J Parkinsons Dis. 2024;14:467-82.
9. Pang SY, Ho PW, Liu HF, et al. The interplay of aging, genetics and environmental factors in the pathogenesis of Parkinson's disease. Transl Neurodegener. 2019;8:23.
10. Singleton A, Hardy J. The evolution of genetics: Alzheimer's and Parkinson's diseases. Neuron. 2016;90:1154-63.
11. Cherian A, K P D, Vijayaraghavan A. Parkinson's disease - genetic cause. Curr Opin Neurol. 2023;36:292-301.
12. Luo S, Wang D, Zhang Z. Post-translational modification and mitochondrial function in Parkinson's disease. Front Mol Neurosci. 2024;16:1329554.
13. Nalls MA, Blauwendraat C, Vallerga CL, et al; 23andMe Research Team, System Genomics of Parkinson’s Disease Consortium, International Parkinson’s Disease Genomics Consortium. Identification of novel risk loci, causal insights, and heritable risk for Parkinson’s disease: a meta-analysis of genome-wide association studies. Lancet Neurol. 2019;18:1091-102.
14. Dulski J, Ross OA, Wszolek ZK. Genetics of Parkinson's disease: state-of-the-art and role in clinical settings. Neurol Neurochir Pol. 2024;58:38-46.
15. Yemni EA, Monies D, Alkhairallah T, et al. Integrated analysis of whole exome sequencing and copy number evaluation in Parkinson's disease. Sci Rep. 2019;9:3344.
16. Abe T, Kuwahara T. Targeting of lysosomal pathway genes for Parkinson's disease modification: insights from cellular and animal models. Front Neurol. 2021;12:681369.
17. Benitez BA, Davis AA, Jin SC, et al. Resequencing analysis of five Mendelian genes and the top genes from genome-wide association studies in Parkinson's disease. Mol Neurodegener. 2016;11:29.
18. Yuan L, Song Z, Deng X, et al. Systematic analysis of genetic variants in Han Chinese patients with sporadic Parkinson's disease. Sci Rep. 2016;6:33850.
19. Guo JF, Zhang L, Li K, et al. Coding mutations in NUS1 contribute to Parkinson's disease. Proc Natl Acad Sci U S A. 2018;115:11567-72.
20. Jiang L, Mei JP, Zhao YW, et al. Low-frequency and rare coding variants of NUS1 contribute to susceptibility and phenotype of Parkinson's disease. Neurobiol Aging. 2022;110:106-12.
21. Jiang L, Pan HX, Zhao YW, et al. Contribution of coding/non-coding variants in NUS1 to late-onset sporadic Parkinson's disease. Parkinsonism Relat Disord. 2021;84:29-34.
22. Araki K, Nakamura R, Ito D, et al. NUS1 mutation in a family with epilepsy, cerebellar ataxia, and tremor. Epilepsy Res. 2020;164:106371.
23. Yuan L, Chen X, Song Z, et al. Extended study of NUS1 gene variants in Parkinson's disease. Front Neurol. 2020;11:583182.
24. Chen X, Xiao Y, Zhou M, et al. Genetic analysis of NUS1 in Chinese patients with Parkinson's disease. Neurobiol Aging. 2020;86:202.e5-6.
25. Bustos BI, Bandres-Ciga S, Gibbs JR, et al; International Parkinson's Disease Genomics Consortium (IPDGC). Replication assessment of NUS1 variants in Parkinson's disease. Neurobiol Aging. 2021;101:300.e1-3.
26. Li H, Yuan L, Yang H, et al. Analysis of SOD1 variants in Chinese patients with familial amyotrophic lateral sclerosis. QJM. 2023;116:365-74.
27. Deng X, Zheng W, Yang Y, et al. Identification of PLA2G6 variants in a Chinese patient with Parkinson's disease. Ageing Neur Dis. 2023;3:9.
28. Guo Y, Sun Y, Song Z, et al. Genetic analysis and literature review of SNCA variants in Parkinson's disease. Front Aging Neurosci. 2021;13:648151.
29. Fan K, Hu P, Song C, et al. Novel compound heterozygous PRKN variants in a Han-Chinese family with early-onset Parkinson's disease. Parkinsons Dis. 2019;2019:9024894.
30. Wang P, Guo Y, Song C, Liu Y, Deng H. PINK1 p.K520RfsX3 mutation identified in a Chinese family with early-onset Parkinson's disease. Neurosci Lett. 2018;676:98-102.
31. Skou LD, Johansen SK, Okarmus J, Meyer M. Pathogenesis of DJ-1/PARK7-mediated Parkinson's disease. Cells. 2024;13:296.
32. Tezuka T, Ishiguro M, Taniguchi D, et al. Clinical characteristics and pathophysiological properties of newly discovered LRRK2 variants associated with Parkinson's disease. Neurobiol Dis. 2024;199:106571.
33. Pejaver V, Urresti J, Lugo-Martinez J, et al. Inferring the molecular and phenotypic impact of amino acid variants with MutPred2. Nat Commun. 2020;11:5918.
34. Li G, Panday SK, Alexov E. SAAFEC-SEQ: a sequence-based method for predicting the effect of single point mutations on protein thermodynamic stability. Int J Mol Sci. 2021;22:606.
35. Cheng J, Randall A, Baldi P. Prediction of protein stability changes for single-site mutations using support vector machines. Proteins. 2006;62:1125-32.
36. Yao LY, Guo JF, Wang L, et al. LRRK2 Pro755Leu variant in ethnic Chinese population with Parkinson's disease. Neurosci Lett. 2011;495:35-8.
37. Imbriani P, Martella G, Bonsi P, Pisani A. Oxidative stress and synaptic dysfunction in rodent models of Parkinson's disease. Neurobiol Dis. 2022;173:105851.
38. Polymeropoulos MH, Lavedan C, Leroy E, et al. Mutation in the alpha-synuclein gene identified in families with Parkinson's disease. Science. 1997;276:2045-7.
40. Deng X, Yuan L, Jankovic J, Deng H. The role of the PLA2G6 gene in neurodegenerative diseases. Ageing Res Rev. 2023;89:101957.
41. Andrews SV, Kukkle PL, Menon R, et al; Parkinson Research Alliance of India (PRAI). The genetic drivers of juvenile, young, and early-onset Parkinson's disease in India. Mov Disord. 2024;39:339-49.
42. Vollstedt EJ, Madoev H, Aasly A, et al. Establishing an online resource to facilitate global collaboration and inclusion of underrepresented populations: experience from the MJFF Global Genetic Parkinson's Disease Project. PLoS One. 2023;18:e0292180.
43. Navarro E, Esteras N. A new mutation in the Parkinson's-related FBXO7 gene impairs mitochondrial and proteasomal function. FEBS J. 2024;291:2562-4.
44. Shadkam R, Saadat P, Azadmehr A, Chehrazi M, Daraei A. Key non-coding variants in three neuroapoptosis and neuroinflammation-related lncRNAs are protectively associated with susceptibility to Parkinson's disease and some of its clinical features. Mol Neurobiol. 2024;61:2854-65.
45. Huang X, Zhao Y, Pan H, et al. The association between LIN28A gene rare variants and Parkinson's disease in Chinese population. Gene. 2022;829:146515.
46. Yu M, Ye H, De-Paula RB, et al. Functional screening of lysosomal storage disorder genes identifies modifiers of alpha-synuclein neurotoxicity. PLoS Genet. 2023;19:e1010760.
47. Long SL, Li YK, Xie YJ, Long ZF, Shi JF, Mo ZC. Neurite outgrowth inhibitor B receptor: a versatile receptor with multiple functions and actions. DNA Cell Biol. 2017;36:1142-50.
48. Fliesler SJ, Ramachandra Rao S, Nguyen MN, KhalafAllah MT, Pittler SJ. Vertebrate animal models of RP59: current status and future prospects. Int J Mol Sci. 2022;23:13324.
49. Edani BH, Grabińska KA, Zhang R, et al. Structural elucidation of the cis-prenyltransferase NgBR/DHDDS complex reveals insights in regulation of protein glycosylation. Proc Natl Acad Sci U S A. 2020;117:20794-802.
50. Harrison KD, Park EJ, Gao N, et al. Nogo-B receptor is necessary for cellular dolichol biosynthesis and protein N-glycosylation. EMBO J. 2011;30:2490-500.
51. Williams LJ, Waller S, Qiu J, et al. DHDDS and NUS1: a converging pathway and common phenotype. Mov Disord Clin Pract. 2024;11:76-85.
52. Xue J, Zhu Y, Wei L, et al. Loss of Drosophila NUS1 results in cholesterol accumulation and Parkinson's disease-related neurodegeneration. FASEB J. 2022;36:e22411.
53. Harrison KD, Miao RQ, Fernandez-Hernándo C, Suárez Y, Dávalos A, Sessa WC. Nogo-B receptor stabilizes Niemann-Pick type C2 protein and regulates intracellular cholesterol trafficking. Cell Metab. 2009;10:208-18.
54. Park EJ, Grabińska KA, Guan Z, et al. Mutation of Nogo-B receptor, a subunit of cis-prenyltransferase, causes a congenital disorder of glycosylation. Cell Metab. 2014;20:448-57.
55. Hamdan FF, Myers CT, Cossette P, et al; Deciphering Developmental Disorders Study. High rate of recurrent de novo mutations in developmental and epileptic encephalopathies. Am J Hum Genet. 2017;101:664-85.
Cite This Article

How to Cite
Download Citation
Export Citation File:
Type of Import
Tips on Downloading Citation
Citation Manager File Format
Type of Import
Direct Import: When the Direct Import option is selected (the default state), a dialogue box will give you the option to Save or Open the downloaded citation data. Choosing Open will either launch your citation manager or give you a choice of applications with which to use the metadata. The Save option saves the file locally for later use.
Indirect Import: When the Indirect Import option is selected, the metadata is displayed and may be copied and pasted as needed.
About This Article
Special Issue
Copyright
Data & Comments
Data
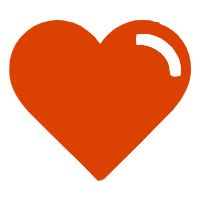
Comments
Comments must be written in English. Spam, offensive content, impersonation, and private information will not be permitted. If any comment is reported and identified as inappropriate content by OAE staff, the comment will be removed without notice. If you have any queries or need any help, please contact us at support@oaepublish.com.